---
This translates into lower operating costs and reduced environmental impact.
A classic approach to superheat control is the use of PID regulation. Unfortunately, when we set ourselves the ambitious goal of running furniture with extremely low superheat, we run up against the limitations of such an approach; liquid leaving the evaporator which implies loss of efficiency, liquid return to the compressor or an unstable system, with a highly variable superheat level.
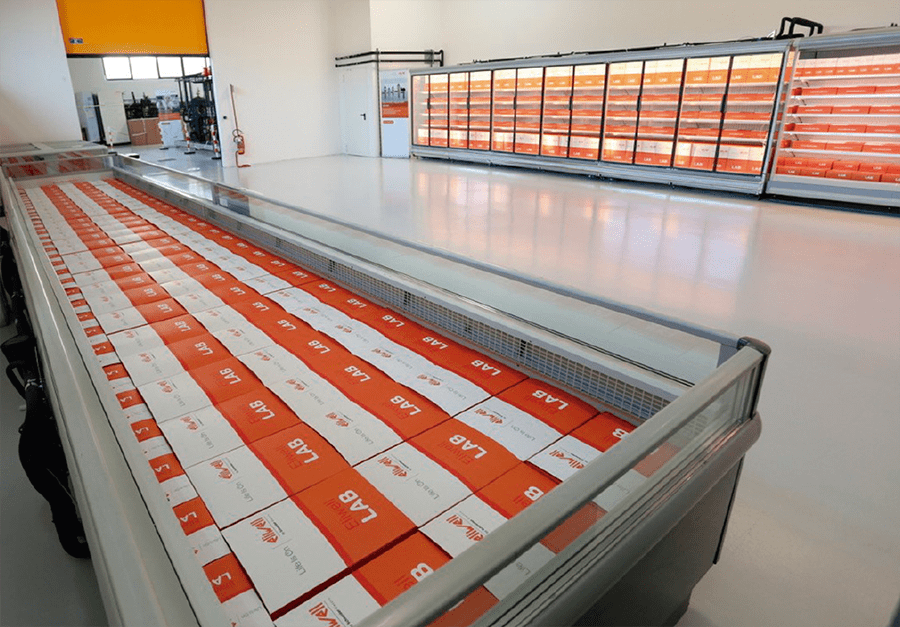
The DOMINO reheating system under DOMINO developed by Eliwell is based on a mathematical model of the evaporator thermodynamic cycle, which tracks evaporator filling, anticipating and limiting excess refrigerant. The controller performs an estimation of the refrigeration cabinet characteristics that takes into account, among other things, any errors or delays in the measurement, non-optimal valve size and refrigerant velocity.
In addition, the DOMINO system is a predictive system, i.e. the model is constantly updated to compensate for changes in the refrigeration plant or cabinet. This allows to obtain a low superheat in all operating conditions with significant energy savings in the installation.
PID ALGORITHM FOR SUPERHEAT CONTROL OVERHEATING CONTROL
The modulating systems of electronic expansion valves are based on superheat values at the evaporator outlet, this superheat depends on the opening degree of the expansion valve, as well as on the absorbed heat.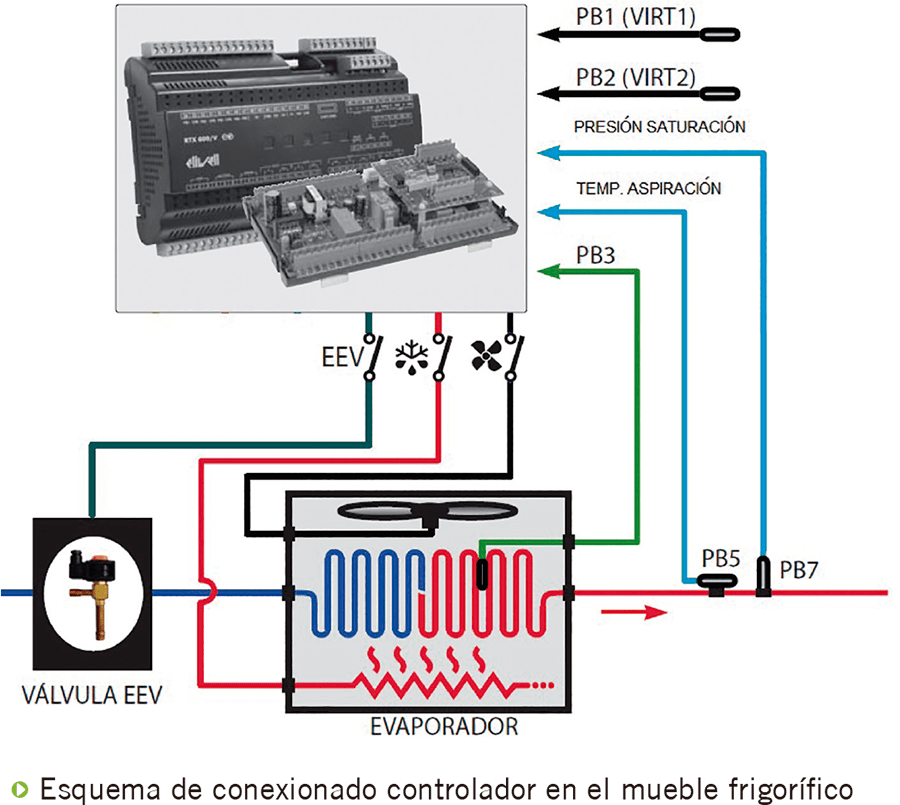
Conventional systems use PID algorithms to decide the opening degree of the electronic expansion valve at a given instant based on the superheat value measured at the evaporator outlet.
The PID algorithm is the most commonly used when there is no knowledge of the process to be controlled, since an acceptable response can be obtained by adjusting only 3 variables. The 3 control variables are those that give name to the algorithm, P - Proportional, I - Integral and D - Derivative.
Usually the first 2 components are used, Proportional and Integral P+I and in some cases the derivative component D is added.
Some factors such as the non-linearity of the expansion valves, delay in measurement and control action and other key factors for regulation such as evaporator filling time or refrigerant speed mean that the PID algorithm does not allow low and stable superheat values to be obtained.
To avoid these oscillations, a higher superheat value is set and the evaporating pressure is lowered to avoid liquid leakage from the evaporator.
LABORATORY ANALYSIS
In order to compare the performance of the PID control with that based on the mathematical model, a series of tests have been carried out with different refrigeration units.
The furniture has been installed in a climatic chamber that controls the temperature and humidity with values of 25ºC and 60 %Hr. For the comparative study, temperature and humidity measurements were taken every minute.
Three flow probes, three return probes and two inertia probes have been installed in each of the refrigerated cabinets. In addition, 2 temperature and 2 humidity probes have been installed in the climatic chamber for its control.
Furniture has been placed in the chamber to simulate the operation of a real installation.
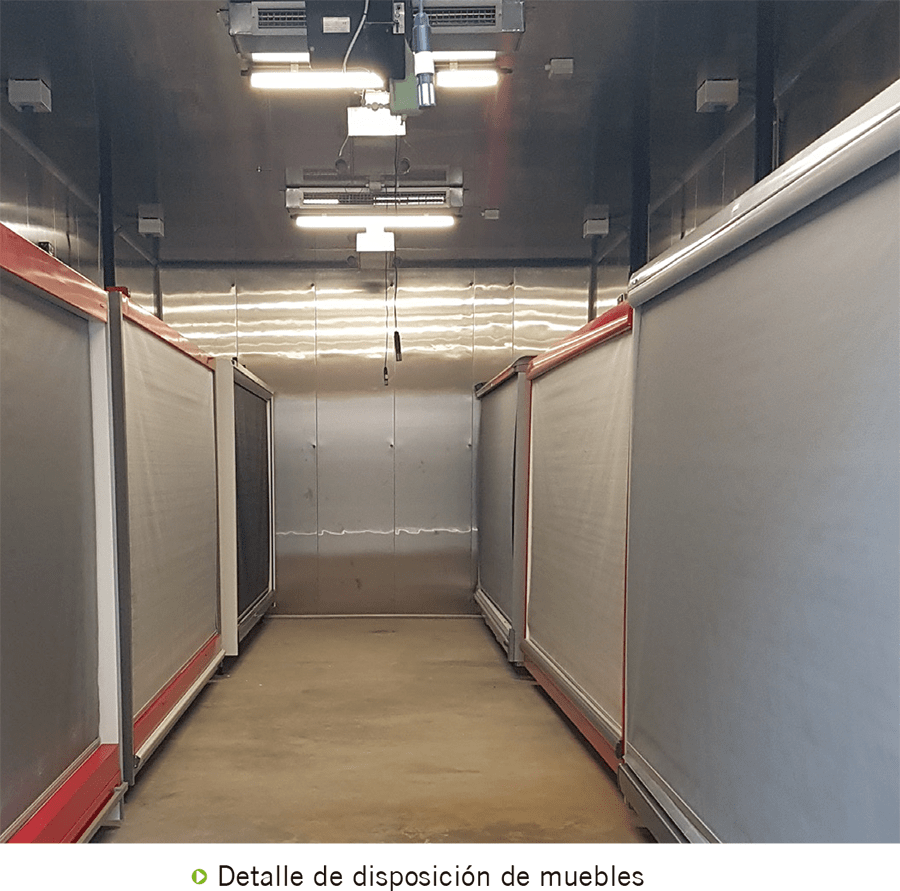
The refrigeration units are connected to a refrigeration unit with R134a refrigerant with speed regulation on the first compressor and on the condensing fans.
The following image shows a refrigeration cabinet where a PID algorithm based control system and the system based on the mathematical model of the evaporator have been installed. The cabinet is located in a temperature and humidity controlled chamber where there are also other refrigeration cabinets in operation.

Multiple ambient temperature probes have also been installed in the refrigerated cabinet, as well as an inertial probe to analyze the temperature behavior with the different options.
The 2 tests were carried out with the same refrigeration cabinet, the same electronic valve and the same refrigeration plant, simply changing the controller control firmware, one with a PID algorithm and the other with an algorithm based on a mathematical model of the evaporator thermodynamic cycle.
During the simulation, several operational tests have been carried out with the system based on the PID algorithm. Specifically, tests have been carried out with evaporation temperatures of -10ºC, -8ºC, -6ºC, -4ºC and finally with evaporation temperatures of -2ºC.
During the different tests it has been verified that with evaporation temperatures down to -6ºC, the controller with PID algorithm correctly maintains the temperature of the refrigerated cabinet and its operation is correct. If, on the other hand, evaporation is increased above this value, the superheat behavior becomes unstable and, in order to avoid liquid leakage, the parameters of the PID algorithm had to be modified without obtaining sufficient stability in the refrigerated cabinet temperature.
During the tests it is intended to maintain a temperature of 0ºC in the cabinet.

The picture shows the superheat value with PID control in the upper part in red and with mathematical model-based control in the lower part in red.
The system with mathematical model maintains the superheat between 5.4 and 4.3 superheat values, while the PID system between 6.1 and 2.5 values.
The picture shows the behavior of the opening degree of the valve. It can be seen how the system based on the mathematical model (below in green) maintains at each instant the right amount of refrigerant needed by the refrigeration cabinet.
With an evaporating temperature of -2ºC and a superheat setting of 4ºC the system with PID algorithm tries to maintain the set superheat value, but as the refrigerant takes some time to travel through the evaporator, there is a time lag between the signal sent to the valve and the superheat value.
On the contrary, the system based on a mathematical model adapts to the value of the time needed to run through the evaporator, allowing to anticipate what is going to happen, so that it injects the right amount of refrigerant with low superheat values and allowing to maintain the temperature of the refrigerated cabinet with an evaporation temperature of -2ºC.
The following image shows the temperature value of the furniture. At the top with the PID algorithm and at the bottom with the mathematical model-based system.
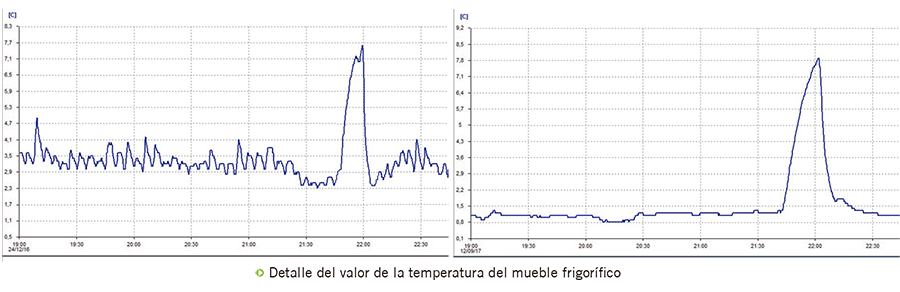
As can be seen, the system using the mathematical model allows maintaining the temperature with average values of 1ºC, while the system using the PID algorithm cannot maintain the temperature within the required limits.
In addition, the PID-controlled system has oscillations in the chiller temperature due to the superheat oscillation and because the PID of the electronic valve conflicts with the PID of the central compressor controller. This means unnecessary compressor starts and stops.
On the other hand, the system based on the mathematical model allows the compressor equipped with a variable speed drive to be kept in operation, with both algorithms tuned to each other.
REAL ANALYSIS WITH R744 IN TRANSCRITICAL TRANSCRITICAL PHASE
Let us now look at a practical application where the solution based on the mathematical model of the Eliwell evaporator has made a difference.
We chose a medium-sized installation, a 1,200m2 supermarket with 9 refrigeration cabinets, a positive temperature chamber, 3 freezer cabinets and a freezer chamber.
The TranscriticalCO2 booster control unit consists of 3 compressors for positive temperature and 2 compressors for negative temperature. The electronic control of the refrigeration plant was carried out with an EWCM 9000 PRO / CO2T DOMINO unit.
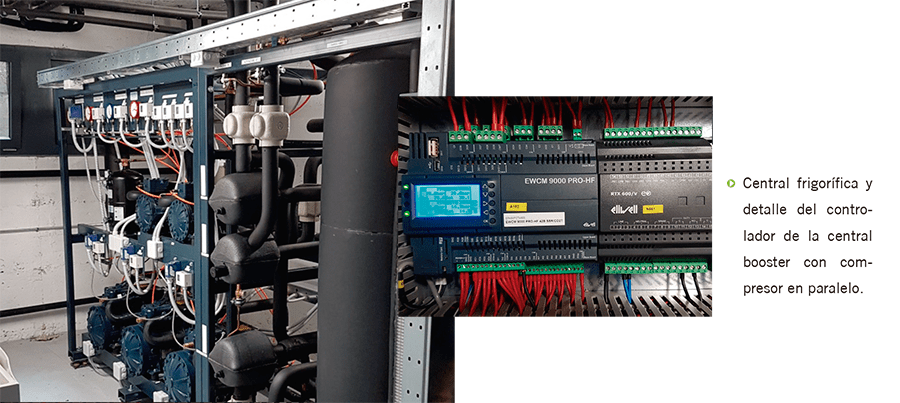
In this plant, the evaporation pressure has been increased from 25 Bar to 30 Bar, achieving an energy efficiency of more than 10%. The possibility of further increasing the pressure up to 32 Bar can mean at least another four percentage points of consumption optimization, ensuring not only maximum efficiency and sustainability of the solution, but also the quality of conservation.
With traditional PID control, it can be seen how the working capacity of the compressors in the refrigeration plant shows typical oscillations of this type of control, producing activations for short periods of time.
With the DOMINO system, however, it is possible to verify that the plant operates at constant load, significantly reducing the number of compressor interventions.
At the same ambient conditions, the number of compressor starts and stops is reduced from 50-80 to only 10 per day, allowing the evaporation rate to be increased to 30 Bar (-4ºC).
In addition, by equipping the system with Eliwell's TelevisGO supervisory electronics and thanks to its algorithm for analyzing the temperatures of furniture and chambers, it would be possible to further increase evaporation up to 32 Bar (-2ºC).
One of the improvements in the operation of the mathematical model system is that it avoids evaporator draining and allows better maintenance of the refrigeration cabinet temperature.
The following images show the behavior of 3 pieces of furniture in ON-OFF and modulating operation.
In red the values of cabinet 1, in blue those of cabinet 2 and in green those of cabinet 3.
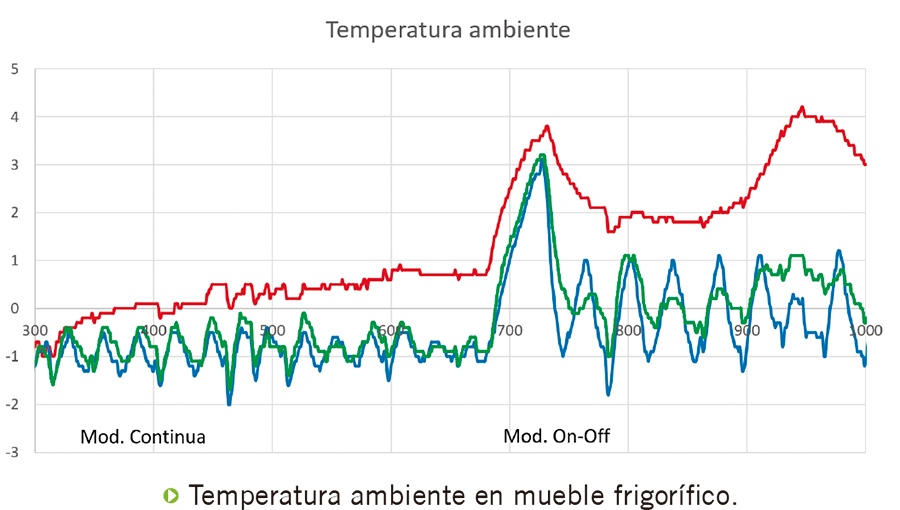
The image shows the stability of the ambient temperature in the refrigerator cabinet itself.

In the previous image, it can be seen that the classic PID superheat control systems enter in certain operating conditions, in resonance with the evaporation control of the refrigeration plant. This results in superheat oscillations and therefore in risk of liquid return, to solve this behavior the superheat set is increased.
The system based on the mathematical model of the evaporator allows the use ofCO2 with superheats between 4K and 2K, seeking maximum heat exchange in the evaporator, without liquid return to the compressors.
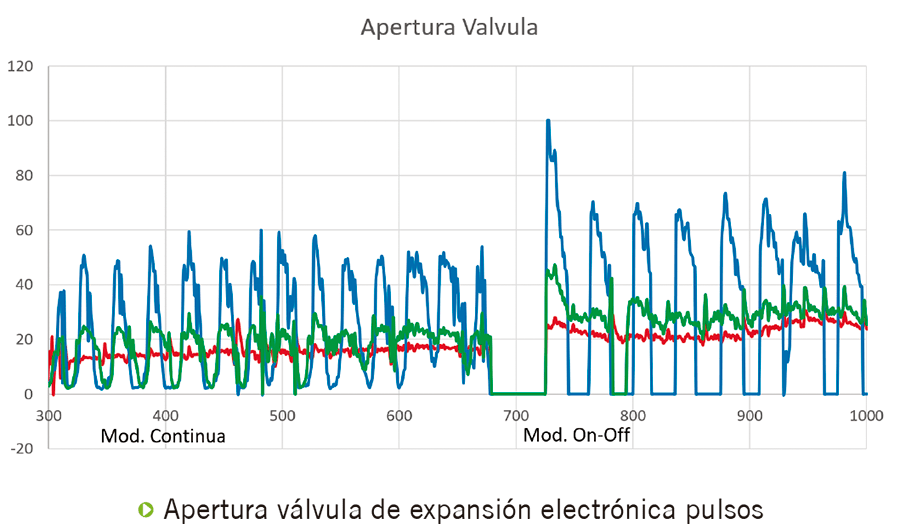
The picture shows the operation of the expansion valve. It should be noted that the valves used are of the pulse type, since they are the ones that allow a quick response in the control of liquid injection into the evaporator.
As shown in the image, the classic PID controls vary their opening percentage from 0% to 100% without obtaining stability in the superheat and penalizing the thermal exchange.
On the contrary, the regulation based on the mathematical model of the evaporator obtains a continuous modulation, with constant mass flow, allowing a tuning between the evaporator control and the control of the refrigeration plant.
ENERGY ANALYSIS IN A REAL INSTALLATION REAL INSTALLATION
The energy consumption of a positive power plant of a real installation has been monitored. This analysis is based on the work methodology according to the standards developed in the international measurement and verification protocol developed by the EVO savings verification organization.
The main objective of this analysis is to verify the energy savings obtained with the installation of the mathematical model based device with superheat at 4K and evaporation during the night at (-2.5ºC) and during the day at (-4.8°C) versus a PID controller with superheat at 10K and evaporation of (-10ºC).
The plant's energy consumption data are shown below.
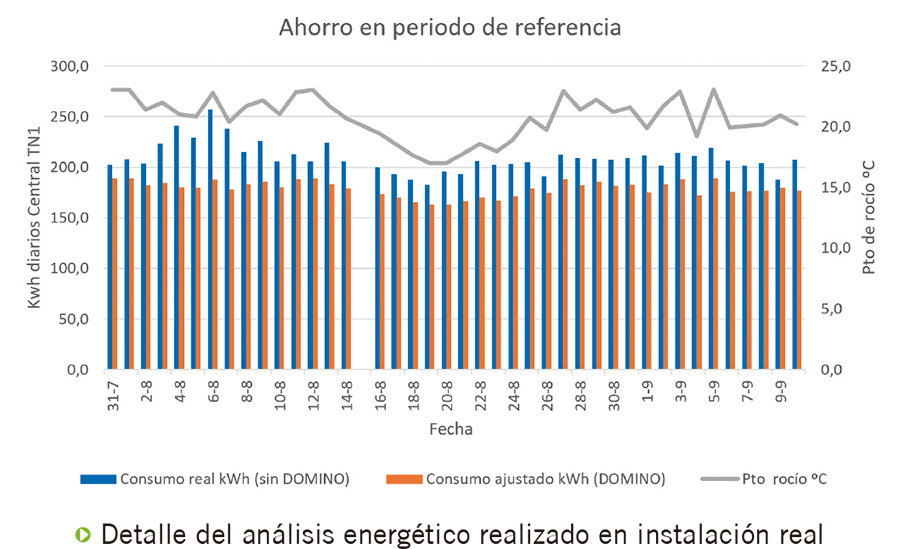
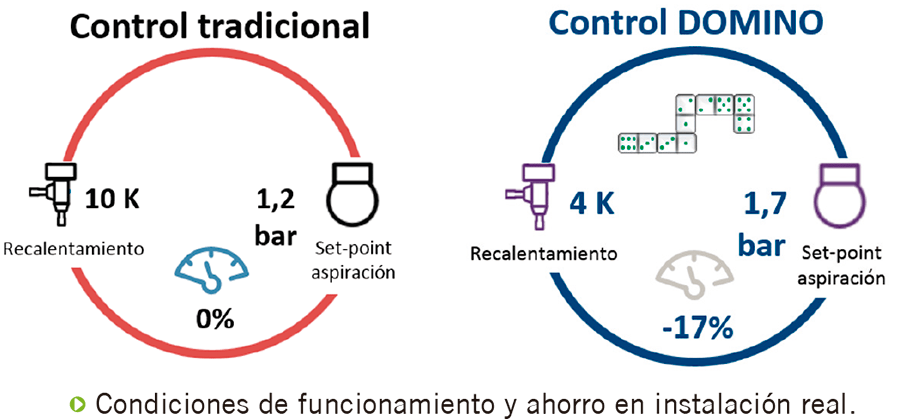
CONCLUSIONS
Traditional systems with PID algorithm can generate liquid outflow from the evaporator or make the system unstable, with time-varying superheat values.
On the contrary, with the system based on the mathematical model of the evaporator, low superheat is obtained in any operating condition with significant energy savings in the refrigeration plant.
In the same way, this system provides stability in the temperature of each refrigerated cabinet, due to the fact that the superheat value is more stable (obtained with pulsed electronic valves for a faster response speed).
This increase in evaporating pressure stability means a reduction in the number of compressor start-stop cycles, which is beneficial in terms of maintenance and increases the compressor life cycle, reducing plant management costs.
It must be remembered that each installation has a stable, ideal minimum superheat, which depends on many factors, including those external to the regulation. The algorithm based on the mathematical model of the evaporator manages to find this minimum value and set it at a very low value, close to 4K.